We shape our buildings and afterwards our buildings shape us.
Winston Churchill
Overview
After food and labor, the biggest factor affecting our swarm’s structure is our resources—our supplies of materials and energy. In the mid 1800s those resources started spiking during the third wave of our industrial phase change—namely, mass production. Then in the late 1800s they started spiking even more during the fourth wave of our industrial phase change—namely, mastery of hydrocarbons, electricity, and magnetism. Those two waves helped lead to big changes in our supplies of food, labor, materials, and energy. That happened partly because of ‘stigmergy’ and ‘recursion.’ Both capture the idea that whatever we make affects what we can next make. Those two swarm-physics behaviors help suggest how, or whether, our supplies of materials and energy might change in the near future.
The King’s Stigmergic Argument
It’s 1851, Britain is top dog on the planet, and it wants to bark. It’s the planet’s first industrial nation, its first half-urban one, and its sole superpower. It speaks for Canada, Australia, New Zealand, and, in effect, India. It owns or leases chunks of Asia, Africa, South America, the Caribbean, and many Pacific islands. It’s just ended its overseas slavery and its forced press-ganging, and is soon to end its penal slavery—although it’s still selling drugs at gunpoint in China (and would continue to do so until 1917). It’s given up being the planet’s biggest slave trader to become the planet’s biggest drug runner. It leads the English-speaking world, so William Shakespeare isn’t just an important writer now, he’s the world’s premier literary genius; Isaac Newton is the very definition of scientific genius; and Walter Scott is the world’s first international best-selling novelist. London, its biggest city, is now the biggest city in the world. With its exports unmatched, its sea power unequaled, and its military might unparalleled, its prestige is immense. So it’s just invited 77 nations, colonies, and principalities to show off their wares alongside its own. They’re all to be displayed that summer at a Great Exhibition, the first ever world’s fair. That’s to be but one more proof that Britannia would forever rule not just the waves, but the world.
The Exhibition, sprawling across 19 acres of Hyde Park in London, is to be housed in the purpose-built Crystal Palace, which is itself proof of Britain’s apparently unchallengeable industrial might, since it’s made with over 4,000 tons of iron, and almost a million square feet of glass. So that summer, as six million visitors gawk at wares from across the planet, most make fun of the paucity and plainness of the United States exhibit. The Times sniffs that “The most popular and famous invention of American industry, is a pistol which will kill eight times as quick as the weapon formerly in use.... Washington does not hesitate to pay about three times as much for cavalry pistols as England pays for infantry muskets.” Ahh, those silly yanks.
Britain has no idea that it’s about be blindsided by a ragtag ex-colony. The United States would soon trampoline onto the world stage with a new production method. It would be a method that for a time Europe would call ‘the American system.’ It would be a method based on a new idea—swappable parts. It would be a method that we today call mass production.
Well, that sounds like a yarn with plenty of rips to be snorted and swashes to be buckled. But that’s not how it went at all. Instead, it was our usual story of fear, confusion, and muddle. The method was indeed real, and it did indeed have those effects, but it was neither new nor American. It was French.
Back in 1763, France had lost a big war against Britain. Humiliated, it had sworn revenge. Of course, every defeated country swears the same thing, but this time a new way of thinking—we today call it ‘science’—was spreading in Europe. France’s young artillery makers vowed to turn gun-making from an art into a science.
Long before Mao said that power grows from the barrel of a gun, every French general knew that happiness was a warm, well-made gun. They all wanted guns whose parts they could swap in the field. What they didn’t have was the skill to make them. Nobody did. Everything, whether as small as a pin or as big as a warship, was handmade—and usually badly. So while French cannon at the time carried an ornate brass motto (Latin for ‘Last Argument of Kings’), that didn’t stop them from blowing up every now and then. Making parts swappable would mean making them to high precision, but standardized parts were unknown. No two parts were the same, so no part could replace another.
However by 1785, in Paris, Honoré Blanc, a French gunsmith, showed the results of years of work to a few VIPs. Amazed, they watched him dip into bins of parts and assemble them into gunlocks. It didn’t matter which part he picked up because he had painstakingly filed them down to fit templates. Each one was the same. By 1790, with state support, he had started a workshop, built machines, and trained helpers. He then gave another demo, at which he assembled about 1,000 gunlocks from bins of parts. That stunned everybody. By 1801, when he died, he had shipped 11,500 gunlocks. By then, about one in every 20 new French guns had swappable parts. So by 1801 France, not Britain, had the first precision-parts manufactory system on the planet. Perhaps Britannia would soon lose power to Marianne.
So France went on to become our first mass-producing country, right? Nope. Making gun parts the same and to high precision made them more swappable, but also more costly. The new guns cost twice as much as the old ones. Plus, adopting them wholesale would mean disrupting our age-old piecework system, where only skilled gunsmiths made guns. So riots might follow. Also, if the unskilled could start making guns—after all, they would only have to follow templates—the state might lose its monopoly on guns. That was unthinkable. All in all, it was too much of a change for the state’s grayer heads. So although the idea found favor in France before the Revolution there, it was buried not long after. France soon forgot how to make precision parts. Ahh, those silly frogs.
Meanwhile, Marc Brunel, a French machinist, and a Royalist, fled France during its Revolution. He first landed in the United States, then later settled in Britain. He had heard that Britain’s Navy valued ship’s blocks. (Intricately carved pieces of wood that worked as 3-for-1 pulleys. Sailors used them to raise and lower sails, move cannon, and so forth.) But blocks were handmade, so quality was mixed, supply erratic, and costs high. Brunel wanted to turn block-making from an art into a science.
His scheme wasn’t just about precision parts but also about dividing our labor when block-carving into stages. Each stage would have its own precision-made carving machine, with all machines powered by one steam engine. However, when he presented his plan to the heads of Britain’s chief block manufactory, they laughed. They said that their system couldn’t be bettered. So he patented his idea in 1801, got together with a naval machinist, who controlled one of the Navy’s steam engines, and another machinist, who had taught himself how to make precision tools, then started a block-making factory. By 1805 it had one 12-horsepower steam engine, ten unskilled hands, and 43 all-metal, high-precision woodcarving machines. Soon it was making 130,000 blocks a year—more than Britain’s six leading block manufactories put together. So by 1808 Britain, not France, had the first mechanized assembly line on the planet.
So Britain went on to become our first mass-producing country, right? Nope again. As in France, Britain’s artisans had zero interest in change. For instance, millwrights wouldn’t suffer any job to be done by the unskilled. They had to do it themselves, no matter how simple. (And why not? They got paid double for overtime.) Riots might follow any change. The Navy, too, mostly could care less. It was already top of the military league. Plus, newfangled ideas might upset their paymaster jobs—not to mention their control over lucrative procurement contracts. Only its heavy need for blocks during Britain’s long war with France, after the Revolution there, had forced it to support the new factory in the first place. Then in 1815, disaster struck—peace broke out. Demand for blocks fell. Soon, Brunel was deep in debt. He, and his family, were thrown in jail. Britain soon forgot how to build assembly lines. Ahh, those silly limeys.
The tale of mass production might have ended there, except that in the crowd at Blanc’s 1785 demo was an idle foreigner, Thomas Jefferson. He was then in Paris as his country’s ambassador, but he was at loose ends because he was being ignored. Only the Prussians thought that his newborn country might survive long enough to bother trading with. The rest of Europe thought it was doomed.
And quite rightly, too, because it was just a little ex-colony. Its war to get out from under Britain’s thumb had ended just two years before—and it likely would have lost that war had France not helped. Its Constitution was still two years away. Connecticut and Pennsylvania were at war. North Carolina was at war with someplace called ‘the State of Franklin.’ Massachusetts and New York were squabbling. So were New York and Virginia. Kentucky was drifting into Spain’s orbit. Besides, everyone there lived within just 50 miles of the ocean’s tidewater, so naval assault from Europe could reach everyone. Plus, the whole population was just 2.5 million, which was four times smaller than Britain’s, and little more than just twice London’s alone. And, as is usual after a war, everyone there was broke.
So Europe saw the jerkwater settlement, with its natives and slaves and wild animals, as an unruly British splinter. Obviously this 100-mile-wide, 1,000-mile-long wedge of forest along the northeast coast of North America would soon be swallowed by Europe’s real powers: Britain and France. Or maybe even sickly Spain. Or perhaps even feudal Russia. The shaky little union knew that conflict was bound to come with each of them (and it did). It also had to find money to keep buying off African pirates, who were attacking its Mediterranean trade. (It would soon go to war with them, too.) That’s to say nothing of its native wars. And its slave revolts. It needed guns.
So in 1785, Jefferson’s eyes must have bulged as he watched Blanc put together one gunlock after another. He then tried to get Blanc to emigrate. Blanc, tired of the political infighting for funding in France, agreed. But Jefferson’s government, as wobbly as a newborn foal, couldn’t raise the money. So when he got back home in 1790 he kept pushing the gun-making idea. Then in 1792 voices in France started singing about “ferocious soldiers who were coming to slit the throats of your sons and wives.” The guillotine fell and the tumbrils rolled. The French Revolution had come.
Everyone panicked. Fear of war with France spread. All Europe armed. Weapon prices soared. The infant United States was petrified. It didn’t have enough skilled hands to make all the guns it needed. Nor did it have the money to buy all the guns it wanted. It didn’t even have a government strong enough to squeeze such money in tax. Plus, of the few guns it could afford, fetching them from Europe was dicey since the French Navy was making threatening noises everywhere. It then started fighting an undeclared war at sea with France.
In 1801, the year Blanc died in France, and the year Brunel patented his idea in Britain, Jefferson became president of his small, scrappy—and scared—little country. He then approved its first loans and grants, trying to induce gun-making at home. Lacking many skilled hands in a land of hicks, the idea was to make do with unskilled hands by aiding them with precision machines.
Cost was high, but so was fear. Yet even that fear wasn’t enough because the country soon expanded so fast that it was land-rich, and thus labor-poor. Anyone with a grubstake could head west and homestead. However, as the government money flowed, and flowed, and flowed, machinists there formed a reaction network, much as early steam engine builders in Britain had. After importing, copying, or stealing ideas from Britain and France, decades of failure went into figuring out how to put Blanc’s precision-parts idea together with Brunel’s assembly-line idea to make it work without many skilled hands. That system would develop into mass production.
Thus, in 1851, at the Great Exhibition, while the riffraff tittered at the United States exhibits, the powerful few, in both Britain and France (and, later, Germany and Japan, then elsewhere) didn’t. They were struck by the quality of those new locks, watches, harvesters, and, above all, guns. After two generations, they had forgotten both Blanc and Brunel, so they couldn’t see how guns could be so well made. So over the next few decades they reimported from the United States the ideas, methods, and tools behind mass production.
How then did the United States do what it did, when both France and Britain failed? Well, add to our usual story: accident and emergency and coincidence, and a strong drive to succeed when all about them failed. But above all else, the United States needed a welcoming attitude to machines, just when precision machines were becoming available after decades of work in France on swappable parts, and decades of work in Britain on steam engines.
Just as with the steam engine, machines and machine tools were only then just barely reliable enough—and powerful enough—and widespread enough—and cheap enough—to begin to replace some of our labor. Without the precision tools and power sources that Europe was then busily creating—and which the United States was just as busily buying, copying, smuggling, or outright stealing—mass production in the United States might well have been impossible.
We can dream all we want to, but invention would be impossible if we always have to start from scratch. To say it another way: things we’ve already built both suggest and constrain what we can next build.
We aren’t unique in that. Termites, like all colonial insects, build things that way, too. Entomologists might call that stigmergy (‘self-building’). Termites are tiny, almost blind, and nearly brainless. Yet some of their species build nests that support millions of them. Such a nest lets them farm fungus, protect their eggs, and divide labor among themselves—and they build such nests without any blueprints to follow or bosses telling them what to do. Instead, they work bit by bit, letting whatever they’ve already built implicitly guide what they next build. Thus, a termite might drop a ball of clay or dung in the nest, and that ball might incite another termite to add another ball. Soon there might be a pile. The bigger the pile, the more it attracts more termites to add to it. Over time, they might thus build an arch, then perhaps a tunnel. They thus change the world around them, then react to that change. Each change alters what they do next. That lets them work together without needing to be aware that they’re doing so. They can thus build large and intricate things without bosses, or any idea of what they’re doing.
We’re similar. We always build on our inheritance, whether we wish to or not. We hand off tasks from one person to another, one firm to another, one country to another. Invention would be impossible if every inventor had to pay for all the inventions of everyone they depended on.
However, that needn’t be how we prefer to see ourselves. Just one year after the Great Exhibition in London, the New York Times crowed that “we see nothing irrational in the hope of a more dazzling future for [our] race than imagination has yet ventured to outline. Not a continent, a half-globe, but the world—shall be ours.” The next July, the United States opened its own Great Exhibition, in what is today Bryant Park in Manhattan. Housed in a new, even larger, Crystal Palace, it was to be but one more proof that Columbia would forever rule the world. Ahh, those silly yanks.
Amalthea’s Recursive Horn
Mass production is as important a trigger of our industrial phase change as the steam engine and the railroad. Before it, we didn’t have factories; at most we had manufactories—places where some of us got together to make things by hand (that’s what ‘manufacture’ means—‘make by hand’). But aside from big things, like wooden warships, which needed large teams, mostly one of us made one thing, like, a gun. That limited how many guns we could make, and how fast we could make them. It also limited how cheaply we could make them, and how reliably we could make them. Also, since there was no such thing as a spare part, gun repair meant that users had to be near makers (as opposed to suppliers). Further, even if many of us wanted guns, every town had many more shoemakers than gunsmiths because making guns was far harder than making shoes. So guns were more like sculptures or paintings than shoes or stoves. (Today, software production is in many ways similar.)
Before mass production, lots of us could make guns, but none of us could make lots of guns. After it, we could make lots of a lot of physical things, from guns to warships. If we wanted something badly enough, the maker of that thing could be anybody, or a network of any number of anybodies, and they could be anywhere, or a network of any number of anywheres. Thus, the speed of making such things could keep rising. The number of such things could keep rising to meet, then even exceed, demand—and so could their variety—and their reliability. Plus, their cost could keep falling. (Maybe one day the same will happen to software, and many other things that are largely mental, not physical.)
That shift was a vast production phase change. But we took a while to get there, because, as usual, we didn’t know what we were doing, nor where we were going, so we bumbled along in fits and starts. We took a century to meander from Brunel’s first mechanized assembly line in 1808 in Britain to Ford’s first Model T in 1908 in the United States. Then we took another century, to 2008, before Fanuc in Japan finished its first fully automated factory—where each month, completely unsupervised robots built 3,000 more robots. We seem to be nearing a time when we’re completely cut out of our own (physical) production system. (And perhaps one day, our mental production system?)
How did all that happen?
First, what exactly is ‘mass production’? Unlike the steam engine or the railroad, mass production isn’t a thing or a network but a process, and we often confuse it with several related processes. We often call something ‘mass-produced’ if we make it in volume. But that’s an effect, not a cause. Over two millennia ago we stamped coins by hand, but so what? That didn’t affect how we could make other things. Much later on, we made coins by building a coin-stamping machine and powering it with a steam engine, but that wasn’t a new kind of production, either. It was still just volume production. Instead of one of us banging on metal with a hammer, a machine banged on lots of metal with lots of hammers.
Mass production is a special kind of volume production that combines several ideas, one being division of labor. For example, suppose we’re making pins. One pin-maker might take one day to make one pin. But if ten pin-makers work together, passing partly made pins between them, with each doing only one specific task over and over, they might make 48,000 pins a day. However, that, too, is just another kind of volume production.
While we could make more pins by dividing our labor, those pins needn’t be precision-made. On the other hand, we could make precision pins, but needn’t divide labor to do so. For instance, we might pour metal into precision-made pin-shaped molds. That’s how we discovered how to make lots of identical clay pots—instead of sculpting clay, we first liquefied it, then poured it into molds and let it set before firing it.
Further, coins, pins, pots—such things aren’t themselves machines. They don’t have parts that have to fit together at high precision for them to work. But guns do. So neither working together in a manufactory, nor powering that manufactory with steam engines (or slaves, horses, waterwheels, or some other power source), nor dividing our labor, nor sequencing our divided labor to form an assembly line, nor making precision parts alone need give rise to an entirely new (physical) production system. That happened only when we put all those ideas together. That’s ‘mass production.’
Once we figured out how to use templates to make precision parts, one part was the same as any other. So we could put them in bins and use whichever came to hand. Also, once we divided our labor when making them, we could make them in a sequence of small and specialized steps on an assembly line. We could then standardize each of our specialist tasks on that line. We could also do the same thing when putting the parts together. Once we did that, we could make specialized tools to help us do each of our specialist tasks, either to make parts or to put those parts together. Then, we could power those tools, and just guide them. That gave us a network that could both make and put together many copies of anything, even if it’s made of precision parts that have to work together to do something.
That magician’s apprentice was pretty nifty, but it wasn’t the truly unusual step because all it could give us was piles of copies of anything. The key step was what happened next: making machines not to make just anything—like coins or pins or pots—but more machines. That closed the feedback loop.
Since the network that we were building was made of tools, and each tool was itself made of precision parts—and the network could make and put together things made of precision parts—we could use the network itself to make and put together each tool in the network. Over time, that gave us assembly lines feeding more assembly lines, which fed still more assembly lines. Then, wherever we still did something by hand, we could start looking for ways to instead do it by inventing yet another tool—whose production we could then fold into the network itself. In time, we folded more and more parts of the network into the network—until there was nothing left to fold. In the end, we got parts, and tools that made and put together those parts—to make yet more tools, which made and put together yet more parts. Everything the network needed, the network made.
That wasn’t just a magician’s apprentice. That was magic.
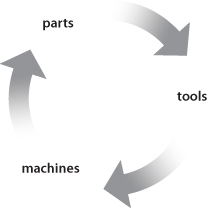
Tools Make Machines Make Parts Make Tools
That kind of self-referencing network isn’t unique to us. Computer scientists might call the network made by all such tools and parts recursive; it feeds on itself (it recurs in its own definition). That property of self-reference is central to all our self-helping production systems. Autocatalysis happens when a reaction catalyzes itself, so it helps itself continue. But that’s just a special case of synergy, which happens when several reactions catalyze each other, so that, together, they help themselves continue. Stigmergy happens when several reactions interact to make things in the world around them that then go on to catalyze more such reactions, so that one thing can help several things—or several things can help one thing—or several things can help several things—continue.
Although mass production was a new process for us, it wasn’t an entirely new idea. Greek myth had long ago claimed that Zeus gave Amalthea, his childhood nurse (possibly a goat), a goat’s horn full of fruits and grain. It was a horn of plenty, which could never empty. Thousands of years later, and piece by piece, we finally made one, without quite realizing what we had done. At first, it was as big as a city block, and much cruder and slower than Amalthea’s horn, but out of it came much more than food for Greek goatherds.
Of course, we didn’t figure out all the pieces, nor how to mesh them together, by planning it all out ahead of time. It was far too vast. As usual, it happened in stages, and by accident.
Here’s how: As our cities exploded, some of us realized that there was so much money to be made that pressure just grew and grew for us to find ways to keep dividing and simplifying and replacing our manual labor. In times past, that couldn’t happen because, over time, wages would rise as the local labor supply dried up. But now, wages didn’t need to rise because, with the railroad, then steamship, there was always more labor flooding into the city. Mass labor remained so cheap and replaceable for so long that factories could just keep expanding as needed. In the long run, strikes against low pay for tough jobs only meant more pressure for more machines to replace more labor. That labor wouldn’t be there in the first place were it not for the push from the rising number of machines (or, earlier, sheep) kicking us off the farm, plus the pull from the rising attractions of the city over the farm. But the machines already in the factories meant that our labor became easier and easier to augment, displace, or replace.
So mass production took shape as cities grew. But cities wouldn’t have grown so rapidly had the machines not been there in the first place. So mass production happened just when we could build machines to begin to replace ourselves, and we had enough labor in one place to begin to want to do so.
However, while mass production would, in time, make many things faster and easier to build, easier to repair, more reliable, and far cheaper, why would any of us bother, if at first those things cost too much for many of us to buy? After all, at first, the machines to make them would themselves cost a lot. Just figuring out how to build them in the first place took lots of time and money. So, sure, we could easily use bits and pieces of the mass-production idea to make more nails, barrels, knives, axes, swords, and such—and, of course, pins, pots, cloth, flour, and the like—but what about more intricate things: like locks, clocks, sewing machines, harvesters, and such?
We might make small numbers of such things, but to bother trying to make them in bulk we needed more than mechanical engineering; we also needed financial and managerial engineering. Without more new inventions—like steam-printed penny newspapers to advertise them, mail-order catalogs to order them, installment plans to sell them, railroads to spread them—factories to make them would have been pointless. Also, regardless of mass production, as all volume production grew, cities grew, so things like department stores and shop windows sprang up. Without all such things we wouldn’t see, and thus wouldn’t know, what to buy.
But then, after a while, a strange thing happened: cities grew so big that demand grew so high that factories grew so large that supply began to outstrip demand. Suddenly, producers could make more stuff than consumers could use. Companies then had a wholly new problem: How to excite demand for things we didn’t need? A whole new field was born: emotional engineering. Management and marketing began to take over. Mere advertising started turning into sheer manipulation.
That didn’t happen all at once. As in all stigmergic reactions, we had to do one thing before we could do another thing. It’s simpler to work out how to make lots of iron or cloth or pots in Britain (that’s not mass production) than to mass produce guns in the United States. But guns then made sewing machines possible. Sewing machines then made harvesters and typewriters possible, which made bicycles possible, which made cars possible, and so on. Guns germinate steel foundries.
Once we ironed out the problems standing between us and mass production, we steeled ourselves for a future where our entire production cycle would go recursive. The strength or cleverness that we needed to build both massive and intricate things started flowing from our hands to our machines.
After many millennia of no real change, the value of manual labor began to fall. What’s the point of a blacksmith’s heavy muscles when a steam-powered trip-hammer can do the same job a hundred times cheaper? Ditto for a spinster’s nimble fingers when a steam-powered loom can do the same job a hundred times faster. And once we had factories full of machines that could make more machines, they could make any number of trip-hammers and looms at ever falling cost.
That had consequences.
In the midst of a machine explosion, we had more and more hands, but, paradoxically, we started needing fewer and fewer of them to make real any new idea, or design, or process. Changing ourselves is hard, but changing our tools is easy. Well, easier, anyway. Machines don’t talk back (not yet, anyway). If, to make a new thing, fewer of our attitudes had to change, we could make more new things faster than before—once we had the money.
That shortened the distance between idea and reality, and increased the value of concentrating our pots of money. Making more money from money alone became easier and easier. In a few of our countries, but in more and more of their industries, capital began to matter as much as, then more than labor—and folks like Karl Marx noticed. He thought there might be a book in it.
We today often take ‘capital’ to mean money, but that’s only financial capital. For big companies that might be vital in the short run. But in the long run, and whether for big or small companies, capital means all assets—everything we’ve amassed that can help us make more goods and services—power, buildings, factories, machinery, equipment, vehicles, and the like. It’s a form of frozen human energy.
Once upon a time, and not that long ago, capital didn’t change fast, so we mostly paid attention only to land and labor. Nearly every physical thing we had equaled what we—aided by our few tools, like our draft animals and waterwheels and such—could make, given a certain amount of land. The steam engine was our first big tool change, then came the railroad. But with mass production, our toolbox itself started growing recursively. The number and variety of our tools just kept multiplying. In time, instead of being mere aids to us, our tools grew into more equal inputs to our (physical) production network.
Over time, strength, speed, ruggedness, tirelessness, nimbleness, and dexterity stopped being our labor resources in shortest supply. As that change spread in our newly mass-producing nations, we had to draw up new labor contracts among ourselves, thus changing the divisions of labor that we had first introduced between men, women, and children when we had phase changed from foraging to farming. Men grew less valuable mainly as work-machines outside the home. Women grew less valuable mainly as baby-machines inside the home. Children grew more valuable for what they could learn in school rather than in the fields or on the streets. Suddenly, we ‘invented’ childhood.
Before then, and all around the globe, kids were mostly just small adults. For example, in Britain, working age for the poor, which was most of us, began at seven or younger. Kids under ten weren’t barred from mining until 1841. Kids under eight weren’t barred from field work until 1868. Kids under ten weren’t barred from factory work until 1874. Child chimney sweeps weren’t banned until 1875. Until the 1840s, kids seven years or older who were convicted of stealing toys, or a spoon, or a pork pie, could be jailed—or possibly even hung. Until 1857, they could be shipped off to a penal colony for hard labor—in effect, enslaved. Some of those “little depraved felons” went for life. Others went for seven or 14 years. Thus, in 1835 one boy stole 21 umbrellas and was sent to Tasmania for seven years. He was 11. In 1843, another boy also got seven years in Tasmania—he had stolen three boxes of toys. He was nine. At the time, perhaps 100,000 kids roamed the streets of London. Child labor, starvation, disease, near-slavery, harlotry, illiteracy, crime, and bastardy were normal for children. Kids as young as five were bought and sold, and put to work up chimneys, down coal mines, or in the factories and fields. ‘Baby farming,’ which mostly amounted to the paid murder of unwanted infants, was an industry. Such ‘farmers’ might accept many babies at once—supposedly to raise, but often to kill ‘accidentally.’ They even advertised in the newspapers. Dickens didn’t have to make anything up in Oliver Twist. He had to tone it down.
But, over time, in our mass-producing countries, new machines meant that farms, then even factories, no longer needed as many hands. Women, urban women at least, began to buy things rather than make them. Plus, with the increasing resources that those machines supplied, we could, for the first time ever, afford to stuff all our urban kids, even our poorest ones, into schools. Of course, school was as much daycare as it was education, because our kids could no longer be on the farm working with, and learning from, us. Plus, the idea was just as much to beat the lust and rebellion out of them as to teach them new skills. Then, once schools had shaped them, they were supposed to go on to work like little robots in the new factories or offices as laborers or clerks, where we, too, were learning to be robots. The idea was that they wouldn’t even think of stealing from or otherwise molesting their elders. Back then, that was what mass schooling was for. In many ways, it still is. (Perhaps that might change when our tools become more equal inputs to our mental production network. Perhaps not.)
The machine age also changed our ratio of rich to poor by flattening incomes in all our newly mass-producing nations. There, many of us started getting mass amounts of the same things. That was totally new. In all our agrarian nations, even today, most of us live on farms and can’t read. We’re also mostly both young and poor. In our mass-producing nations, though, most of us live in cities and can read. We’re also mostly both middle-aged and well-off. Plus, about as many of us are old as are young. And about as many of us have upper-middle incomes as have lower-middle incomes. So now there was a new thing under the sun—many of us who were neither rich nor poor: we were in a large middle class.
All in all, it was a huge phase change; as huge as the farming phase change before it; and it was just as unplanned, and, once started, just as unavoidable. When we were all foragers, turning into farmers had meant more food per acre. In exchange, though, we were forced into more work per hour. We also got broken teeth, stunted growth, more disease, slavery, and mass war. And women turned into baby machines. When we started turning from farm hands into factory hands, we got more stuff per hour of work. But in exchange, we were divorced from the land that, for millennia, had made us self-sufficient. Rather than work we knew, done on land we knew, at a pace we knew, we worked at an alien place, at an alien pace, with rigid rules, fixed hours, and harsh punishments. We were also subject to distant and mysterious changes in money and trade. So in exchange for more goods per hour of work, we got pushed into work we didn’t control and, sometimes, didn’t even understand. The new work was also robotic—repetitive, single-purpose, and dull. So not only were we bored, we grew over-dependent on having just one skill. Our children, too, were more and more lost to us as they learned new things and went their own ways. So we were in an inherently more unstable state. We also lost fingers, eyes, limbs, even lives to the new machines. Mines were even harder on us. Railroads wiped out whole villages and whole ways of life. Guns and smallpox led to genocide. The new scale of our cities and the new pace of our lives led to new heights of injury, distress, and death—and new depths of crime, misery, and disease. The scale of our wars shot up until millions of us died at a time. Pollution, once a local problem, grew into a global one. And thousands of species died to make way for ever more of us and ever more of our machines.
Trigger Effect
These days, it’s common to talk up how much our world is changing now: Computer this, global that, yada yada. But change compared to what? In 1850, nearly all of us, everywhere on the planet, still lived and worked and traveled and communicated about the same as everyone everywhere had for millennia—then, sproing! In just 50 years, two generations, from 1860 to 1910, and starting in our presently rich countries, our species started into vast changes.
That’s when the huge profits from the exploding cities of our presently rich countries, coupled with the flood of new goods from their mass-producing factories, meant that research and development left the world of idle hobby and occasional insight and became corporate backed. Suddenly, ideas that had been percolating for decades, sometimes centuries, got harvested and applied. That’s when we started into the fourth wave of our industrial phase change as we figured out the physics of electricity and magnetism. That completed a circuit, shocked inventors and investors, and shed new light on how we could make many new tools, from the dynamo to the electric motor to the internal combustion engine. The telegraph, transoceanic cable, telephone, radio, microphone, and phonograph spread then. So did the car, power plant, and light bulb. So did sewing machines, harvesters, typewriters, bicycles, machine guns—not to mention fridges, skyscrapers, elevators, condoms, diaphragms, cameras, anesthetics, antiseptics, dynamite, aspirin, flush toilets, and rolled toilet paper. Each one led to huge changes. (Okay, maybe not rolled toilet paper.) But in all that tumult our single biggest change of all was in our energy supply, for those 50 years were when we really began to consume hydrocarbons.
Made mostly of hydrogen and carbon, those molecules come out of the ground as coal, oil, and natural gas. We had known about them for over seven millennia, but had never used them for much other than caulking, and, rarely, for heat. By the 1860s, though, we started finding more and more uses for them.
For lubricants, animal fats made way for oil. For fuel, hay and wood made way for coal. For heat, wood gave way to coal, then natural gas. For light, animal fats gave way to kerosene, then natural gas, then power grids and light bulbs. For power, the steam turbine and the dynamo led to the power plant and the electric grid. For motion, we replaced hay-driven muscle power with the coal-driven steam engine, then the (usually) water-driven electric motor, then gasoline and diesel fuel for the internal combustion engine. Then, near the end of the period, we used that engine to take to the skies.
From machines started then, many more changes followed in the 1900s. With internal combustion engines around 1880—and electric motors around 1890—we began freeing our bulk transport from horses and oxen, and then even rails. We began to farm areas that we couldn’t have before, and we did so with new machines, like tractors. We also used cheap coal to make electricity in coal-fired power plants. The price of energy fell like a stone down a dry well.
The price of metals also fell as we, for instance, used some of that cheap energy to make cheap aluminum, which drove down the price of copper and tin. Roughly the same thing happened to zinc, lead, iron, tungsten, titanium, and on and on. We also used our newly cheap energy to make stronger alloys, particularly high-tensility steel. Then we used those alloys to build steel ships and railroads and skyscrapers. Our cities exploded even more.
Gasoline and diesel fuel powered everything from cars to trucks to planes to supertankers. Our cities exploded yet more. Trade, and the global division of labor it was inducing, exploded even more. Today, hydrocarbons also power nearly all our vehicles around the globe. About the only exceptions are chemical rockets and nuclear-powered vessels—spacecraft, submarines, and aircraft carriers.
From hydrocarbons also came big changes in our chemicals. We got our first synthetic dyes around 1860. Then, as we worked out the chemistry of those, we were soon making drugs in vats, and on an industrial scale. Out of that came—to name just a few—aspirin, heroin, saccharin. Then, after the 1930s, vitamins—on an industrial scale. Then, after the 1940s, all our antibiotics, and, again, on an industrial scale.
Thanks to hydrocarbons, too, our materials and clothes changed as we got plastics. At first, we molded them to look like the things (made of leather, wood, stone, and such) that we wished to replace; but then we got used to them and started making them look any old how. Then came totally new things: respirators, surgical gloves, plastic wrap, velcro, teflon, epoxy, fiberglass, kevlar, circuit boards, videotape, data discs, electronic paper, plastic TV screens. Out of plastics also came nylons, then polyester, lycra, and spandex—without which we might never have had to endure the disco era.
Cheap synthetic fibers also led to surpluses in older fibers, like cotton, wool, flax, jute. So their prices fell. So cotton farmers switched to wheat, sheep farmers turned to cattle, flax farmers moved to soybean, jute farmers shifted to rice. So those prices fell. As the price of both food and clothing fell, ever more women, no longer as needed for home production of food or clothing, stepped out of the home and into the wage-earning market.
Most important of all, after 1909, our food supply changed a lot as we began using hydrocarbons to make cheap synthetic fertilizers by packaging up nitrogen in the air into compounds that plants can eat. Crop and livestock yields spiked. For example, corn yields in the United States shot up nearly 800 percent in a century. In that country alone, and in just a half-century, all its harvests at least doubled while its number of farmers fell by two-thirds.
By the 1960s, we stumbled on genetic crossings that let our grain crops capture more nitrogen. That, plus our new nitrogen-rich fertilizers, meant that we had, in essence, found a way to turn air into food. Our food supply spiked yet again. For instance, around the world, our rice yields almost tripled between 1967 and 2002. Similarly, our meat supply tripled between 1980 and 2002. We thus fed at least a billion more of us than we could have fed before. Likely, that alone averted worldwide food catastrophe—if not world war.
As of 2012, hydrocarbons were the main ingredients, in money terms, in our food. For example, by then in the United States, we burned at least seven kilocalories of hydrocarbon energy to make one kilocalorie of food energy.
In sum, up to basically yesterday, our energy supply was limited to the solar energy that we could gather with plants. That limited our numbers. Over the millennia, our numbers grew anyway because we kept figuring out clever ways to squeeze a little more food out of the same land. But we still had to do the same work as before because after each small jump we always kept pumping out more babies than we could feed.
We lived in a cave; and while we always chiseled away at its ceiling, we soon bumped our heads up against it again. But once we worked out ways to extract bottled sunshine—captured mostly by plants over the last third of a billion years—we sprang out of the cave as our energy supply jumped to a whole new level. Our species became a trust-fund baby, and we went on an oil-spending spree. From 1900 to 1970, our oil use doubled about every decade. It stuttered after price hikes in the 1970s, then from the mid 1980s to 2008 was again doubling, but more slowly.
Thus, 12 millennia ago, fewer than ten million of us were alive. By 1800, about one billion of us were. By 1930, we jumped to around two billion. By 1975, we crossed four billion. By 2000, we hit six billion. By 2030, we’ll likely be over eight billion. Much of numbers spike is thanks to hydrocarbons. Our machine explosion wouldn’t mean much without an energy explosion to power it.
But while we jumped out of the cave, we didn’t jump far. We just jumped into another cave with a higher ceiling. If we do nothing before hydrocarbons get expensive again, we’ll be right back in the first cave. Will we use our grace period to build a hydraulic jack that will prevent us being squished back down to 1850 energy levels, perhaps with only around a billion of us alive?
We, the Cheapskates
If a future oil shock is almost certain, why don’t we shift our energy sources now, before we have a gun to our head? In a word: Stigmergy. Just like termites building a nest, despite what we might say we want, the energy toolbox that we’ve already built constrains what we can next build.
When it comes to energy, the United States is a good country to focus on because in 2010 it was still immensely rich. Even though fewer than one in 20 of us lived there, its economy was about a fifth of our entire economy. (The European Union was also about a fifth; East Asia—which includes China, Japan, and South Korea—was about a quarter.) It burned about a fifth of all our oil, a quarter of all our coal and natural gas, and over two-fifths of all our gasoline. California alone used more gasoline and diesel fuel than any country on the planet.
In the United States in 2013, a kilowatt-hour of electricity—the amount of energy we burn when we leave on a 40-watt lightbulb for about a day—cost about 10 cents U.S. That price point is the pivot about which all our competing energy sources turn. So far, all other energy sources cost more (at least, in terms of money). For instance, solar power cost at least three times more. And that was not even counting the cost of storing such energy for nights or cloudy days. We haven’t yet figured out how to make big cheap reusable batteries.
Thus, about two-fifths of the United States’ electricity came from the cheapest source: coal-fired power plants. Natural gas supplied roughly another quarter, and nuclear about another fifth. Oil added only about two more percent. That accounted for almost nine-tenths of the country’s electrical energy. Hydroelectric added more than half the rest. The remaining dribble mostly came from biomass (like wood). Wind, geothermal, tidal, and solar were so tiny that they simply didn’t matter. That picture isn’t likely to change much until at least 2030 or so. In terms of electricity needs, if all oil vanished tomorrow the country mostly wouldn’t notice.
But the picture changes a lot when looking at the same country’s total energy use. Oil claimed over a third of that. (Of that oil, almost three-quarters went to transport, and almost a quarter to industry.) Coal claimed a further fifth; natural gas claimed a further quarter or so. Nuclear added roughly another twelfth. Again, that was about nine-tenths of the country’s total energy use (electricity included). But here, only around a third of the rest was hydroelectric. And, again, most of the dribble left was biomass, but increasingly wind. Geothermal, tidal, and solar again simply didn’t matter. That picture might well change before 2030 or so, but, again, likely not by much. Only when our growing numbers, wealth, industry, and—above all—transport, drive up oil prices a lot are we likely to begin to shift our snouts from the oil trough. It’s just too cheap.
The country, with vast distances to cover, spent over a quarter of all its energy on just one thing—transport. It had about a quarter-million planes, and roughly a quarter-billion cars, trucks, motorcycles, and buses. Of all its transport energy, cars, motorcycles, and light trucks together burned 60 percent. Heavy trucks burned 22 percent; planes, six percent. Transport on water, for buses and trains, for farms and so on, all were small.
So were oil prices to rise a lot, here’s what seems likely to happen: No apocalypse, zombie or otherwise. Food and heavy transport wouldn’t go away. Global trade wouldn’t end. Cities wouldn’t collapse—they would grow. Nor would mining stop. Nor would new housing end. Home heating and heavy industry wouldn’t die. Nor would plastics vanish—nor fibers, fertilizers, drugs, and so forth. But recession, and perhaps depression, is certain. So, possibly, is war. And our biggest oil guzzlers—the cars and light trucks—and the suburbs and exurbs that they triggered—would almost surely change. Those urbs, built it on cheap gasoline, may not survive expensive gasoline. Money matters.
Worldwide, in 2010, transport was our single biggest industry; of the ten biggest companies on the planet, six were in transport. Worldwide, in 2012, hydrocarbons (coal, oil, and natural gas) gave us over four-fifths of all our energy, and they have done so since at least 1987. Thus, our biggest resource problem over our next two to three decades probably isn’t going to be giving up hydrocarbons in general, or even oil in particular, but finding a new cheap transport fuel.
However, whether or not we find one in the next decade or so might not matter all that much. Two thought experiments suggest why.
Suppose an alien spacecraft crash-lands tomorrow and in it, besides some dead aliens, we find an exotic energy device—perhaps an oscillation overthruster coupled to a flux capacitor and a machine that goes ‘ping!’ What might happen? In the short term, probably not much. Our stock markets might react quickly, discounting the stocks of many of our current energy companies. Many portfolios might collapse. Many rich foreheads might furrow. Some news outlets might shriek that it was the end of the world. Various recessions might come and go as various of our nations compete for the new tools, and as the stocks of our older energy technology companies slip. But unless the alien tech at once triggered a world war to own it, our toolbox would likely take a decade or more to adjust. Why? We would need time to put new, and cheap, tools in place to use it. And time to minimize its new wastes and dangers, whatever they might be. That is, if we could even understand it—then copy it.
Nor is that scenario completely made up. In 1954, when atomic energy was young, it was just as if an alien power source had dropped from the sky. Some nuclear experts said that it would one day yield electricity “too cheap to meter.” But that didn’t happen—or hasn’t yet, anyway. A nuclear power plant (whether it’s a pressurized water, boiling water, or supercritical water reactor) isn’t magic. It’s just a really big kettle. It’s nearly the same as a coal-fired plant. It boils the same water. It pipes the same steam into the same turbines. It uses the same electromagnets and condensers and transformers, and so forth. It mainly differs only in the fuel it burns—uranium instead of coal.
It wouldn’t much matter whether a power plant’s fuel was plutonium or straw. For example, in 2008 a pound of uranium cost about 1,200 times as much as a pound of coal. But it also yielded about 18,000 times as much energy. Compared to the cost of the plant, any kind of fuel costs only a few tenths of a cent per kilowatt-hour. It’s the cost of the toolbox needed to make use of the fuel that matters. That toolbox includes all the tools we need to find, fetch, store, and burn fuel. We also need tools to convert that fuel’s energy to electricity. Plus we need tools to condition and transport that electricity. And we need tools to monitor and maintain—and pay for—all those other tools. In short, our energy supply depends far more on our toolbox than it does on our fuels.
Further, not only does that toolbox cost a lot, it’s so huge that it’s slow to change. For instance, most of a power plant’s heavy equipment might last for decades. Plus, even when one of its parts—for example, a multi-ton steam turbine—fails, we’re likely to replace it with another multi-ton steam turbine; we won’t replace it with something so different that it won’t fit with the rest of the plant. So all the plant’s part types tend to march lockstep into the future.
Yet further, a power plant is vital—and dangerous. So it’s licensed, regulated, inspected, controlled. So changing any of its major parts may mean large legal costs. Also, once it’s paid for, it will have ongoing costs, but those are small compared to the cost of a complete retool. A power plant might thus last for 40 or more years. For instance, in 2010 in the United States, 24 generating units were over 70 years old.
So no matter how miraculous a new power source may be, at least a decade or more might go by before it could have major effect—lowering materials prices, destroying jobs, and dunking many of our nations into depression (and possibly, war).
On the other hand, suppose that some fraction of our oil supply simply vanished tomorrow, perhaps in some bizarre subsurface fire. What might happen? Again, in the short term, probably not much. Again, our stock markets might react quickly, bidding up the price of oil. Again, many portfolios might collapse. Again, many rich foreheads might furrow. Again, some news outlets might shriek that it was the end of the world. Again, recessions might come and go as various of our nations compete for the remaining oil, or as the stocks of newer energy technology companies jump. We would also have to retool to more quickly use our more costly, or more dangerous, or less portable, options. But unless our sudden energy shortfall at once triggered a world war to own the remaining supplies, at least a decade or more might go by before we really felt its effect—higher materials prices, destroyed jobs, and depression in many of our nations (and possibly, war).
That scenario, too, isn’t completely made up. Big oil price hikes in 1973 and 1979 led to panic, long lines, and recession in many countries. But much of that turmoil in various of those countries might have happened because they tried to control domestic oil prices instead of letting them float. In the United States in 2007-2008, the price of gasoline at the pump jumped by about a third. It was as if the country had lost a big chunk of its oil supply. Some news outlets shrieked that the end of the world was near. But there we just did what we always do when the price of anything rises: We bought less of it. Road travel fell by 67 billion miles a year. The price of fuel-efficient cars rose while others fell. Some of us thought about moving closer to our jobs. Had the fuel crunch continued for long enough, more changes would probably have followed. Perhaps some of us would move—or start losing our jobs. Car pooling would grow. Bus services would be beefed up. Suburbia would begin to die. Or we would build more train services, and fewer highways, and so on. Deciding to go to war instead of doing such things amounts to thinking that it’s better to try to kill a bunch of people now rather than be forced to spend a bunch of money later. Money matters.
Around the planet in 2010, all our non-hydrocarbon, non-nuclear energy sources added up to around 11 percent of our commercial energy supply. About half of that was hydroelectric, and we’ve mostly maxed out on that. Much of the rest was biomass, mainly wood, and we’ve mostly maxed out on that, too. The one growing, almost significant, renewable was wind. Solar was growing, too, but was still too tiny to count. In transport, none of our current choices could compete with oil at current prices. We need more research, and more tools, before that can change. That will take time and money. So for our near future we’ll likely go with what we already know—coal, natural gas, and nuclear for power, and oil for transport. Barring a big disaster, or the arrival of glad-handing aliens, that, plus some smallish changes, paints a likely picture up to at least 2030 or so.
Some Assembly Required
In 2010, counting all our energy sources—straw, oil, plutonium, dams, everything—we burned energy at the rate of about 17 terawatts (that’s 17 thousand gigawatts, or 17 billion kilowatts). However, the sun bathes our planet in an energy flux around 10,000 times larger, less than half of which reaches the surface. To tap that energy we might loft solar panels into high orbit (about 22,000 miles up) so that they always bask in sunlight. They could then beam their collected energy back to us. That might solve all our energy problems for centuries. But while that river of energy is plentiful and free, the knowledge and tools that we would need to get it isn’t.
First off, launch costs are high. In 2011, getting a pound of anything on a shuttle just into low orbit (ignore high orbit) cost at least $21,000 U.S. In 2012, that much money would have bought almost a pound of platinum. So even were the moon made of platinum we probably still wouldn’t mine it. There would be no point.
Only a small part of that cost is due to the physics of climbing out of a gravity well, whether with chemical rockets or otherwise. Most of the cost is due not to physics but to swarm physics.
For one thing, commercial demand for access to space is still small. In space, there’s still no there there. From 1957 to 1999, our species launched about 104 times a year. From 2001 to 2005, we launched about 62 times a year. But of all those launches, only about 19 a year were commercial. From 2010 to 2014, we launched about 82 times a year, but only around 21 a year were commercial—still mostly just TV and phone stuff. With business demand still so low, nearly all our launch systems were still state-owned. So they were still built for attack, defense, spying, and prestige—not energy efficiency. They’re like royal navies with no merchant marines to protect.
To launch rockets, such launch systems used to junk their transports; that’s like driving to the grocery in a high-priced car, then walking home because we had to give the car to the clerk to pay for the groceries. Also, to build those transports, our launch systems had to employ tens of thousands of us. That remained true even when we got into reusable transports. Thus, the United States space shuttle program, before it was shuttered in 2011, employed about 50,000 of us. So each year’s payroll cost about $5 billion U.S., whether or not a shuttle flew that year. From 1981 to 2010 the program’s total cost—counting buildings, support, and research—was $192 billion, or about $6.6 billion a year. In the same period, shuttles flew just 131 times—or about 4.5 times a year. (In 2010, Atlanta International Airport averaged 108 flights an hour—about 4.5 every 2.5 minutes.) Thus, each shuttle mission averaged about $1.5 billion. Compared to that, fuel and other consumables cost nothing—about $60 million per flight.
So, as with our energy grids, launch fuel cost nothing compared to toolbox costs. Those costs can’t fall until we’re launching all the time; but we can’t launch all the time until costs fall. Nor are costs likely to fall anytime soon.
Why? Well, few of our states are space-ready, and our space-ready states aren’t strongly motivated in this matter. And why? Being able to launch, no matter what you’re launching, also means being able to launch a bomb. Being in space when few others are, is like being up a tree armed with rocks while most everyone else is on the ground below. So between states, space-readiness is about deciding who gets to throw rocks, while within a state it’s about deciding who gets to gain from the threat of rock-throwing.
So, despite what the media may focus on, high launch cost keeps others out of your treehouse, which simplifies defense from orbital attack. So instead of the usual paeans to space programs—‘pale blue dot,’ ‘one small step,’ ‘to boldly split infinitives that no one has dared split before’—perhaps we might think of them more like heavily armed trophy wives. They don’t do much, but they look good—and they’re useful whenever the next spat with the neighbors rolls around.
Now consider what it’s like to be an aerospace company. Unlike other businesses, we mostly can’t work for other companies—there’s too little demand—so we have to work for the state. But when we run a trucking firm that’s judged by how photogenic it is on TV, and how easily it can double as a missile deployment platform in an emergency, energy efficiency isn’t our chief worry. Plus, with lives and prestige at stake, many layers of bureaucracy make deciding anything a byzantine affair. And in aerospace, just as in weapons, if we fail at something, the state just ups our budget (because it wants to keep us alive). So what kind of idiots would we have to be to risk our own money trying to make future launches cheaper? (It’s much the same for firms that build nuclear craft—like submarines and aircraft carriers. Such devices have zero commercial use. But if a state fails to keep them alive through all the boring years of peace, it won’t have them when the exciting shooting starts again.)
Thus, while aerospace might look sexy, it’s still mainly a cottage industry, much as gun-making in Europe was before the 1880s. Mass production has yet to reach it. Imagine if every time you wanted to watch a TV show, 50,000 of us had to show up at your house. We would then rebuild your TV set—and the house you’ll watch it in—using hand-built tools—and hand-built parts. Plus, you had to keep us on retainer all year long. And we left with the warning that if you turned it on, one time in 60 it might blow your house up.
That’s the launch cost; what about the solar panels we want to launch? Compared to commodity solar panels, space-ready solar panels are lighter and more efficient—but, so far, such demands switch off the mass-production effect. So they’re hand-made one-offs, intended for military, research, or, at best, comsat use, where money is little or no object. That hugely increases time and cost of manufacture.
In 2013, most commodity versions still captured less than a fifth of the solar energy hitting them. So for each pound of panel, they could only produce, at most, around 22 watts. Also, for every pound of panel we launch, we might need another pound of satellite. (To track the sun while in orbit and to beam energy to the earth, not to mention harden the panel to survive in space.) Plus, we might lose maybe half the beamed energy due to atmospheric warming. So for every pound we put in orbit we might get back maybe five watts.
To simplify the arithmetic, suppose we could somehow amp that up to 10 watts per orbital pound. Suppose, too, that we could somehow drop the price to launch a pound to high orbit to $10,000 U.S. Even then, if we go the mass-produced but heavy and low-efficiency route, we would be paying around $1 trillion U.S. per gigawatt.
That’s not all. What about insurance against failure? Rocket launch is a violent affair—high acceleration, high pressure, massive shaking. Delicate objects have to be packed in such a way that they don’t bend, shear, or break. Then on passing through the ionosphere, the air itself becomes conductive; that alone can short out electrical gear. Then, once in orbit, temperature variations as the satellite passes in and out of the earth’s shadow can cause problems. There’s also the chance of being hit by all the debris already up there, plus the problem of solar storms. So we have to worry about electrical shorts, fuel-line leaks, failed explosive bolts, bad software... it’s a long list. For one reason or another, about one in five of our satellites might fail to deploy.
That’s a potentially big hit, given that even the average comsat costs at least $250 million. Who knows what powersats might cost, since we haven’t built one yet. That (unknown) cost also doesn’t include assembly in space. Nor upkeep in space. Nor the ground station we would need to catch and distribute the beamed power. Nor that station’s power-conditioning equipment. Nor its costs in salaries, buildings, insurance, interest, and depreciation.
There’s also the cost of designing and legalizing a powersat in the first place. For example, we couldn’t beam the power back with lasers—they’re too accurate, so they could double as weapons. Space-weapon agreements ban that. So we would have to use microwaves. But, at present, we’re already using the best microwave frequencies for cell phones here on earth. Figuring out how to get around that will take money.
Nobody knows what all those costs might add up to, but if it’s anything like the space shuttle’s costs, they might add another few hundred billion dollars per gigawatt. That might jack up costs to perhaps $1.5 trillion per gigawatt. Meanwhile, back at home, in 2010 a new coal plant cost something like $1 billion U.S. per gigawatt—1,500 times cheaper.
Of course, if we had to build many such orbital plants in a hurry—maybe, a decade—or go extinct, we probably could do it. It would cost billions per powersat and trillions to figure out, but we would put our best people on it and spend the money (and be willing to lose the lives) and get it done. We know that because two giants fell into a moon race back in the 1960s and more or less did just that. However, as long as there’s no real hurry, it would remain a business decision.
As long as it stays a business decision, even after we work out how to cut powersat costs, and how to finagle the legal and military risks, there would still be financial risk, as with any power plant. A powersat would take years to work out, build, and deploy. Then it would take more years to pay for itself before turning a profit. Meanwhile, investors would be paying the opportunity cost of not being able to use their money for anything else in all that time. They won’t risk megabucks unless they could make gigabucks. Plus, a power plant might last for decades. For all that time its investors would be betting that cheaper options wouldn’t come along and destroy their profit margin.
Nor would they be crazy to worry. In the late 1600s, France, fearful for its navy’s future, planted oak forests. Those matured a century and a half later. But by then, they were useless for their intended purpose—because the steamship had arrived. More recently, on launch in 1990 the Hubble space telescope had ten times sharper focus than our best ground-based telescope. By 2010, its total cost, counting upkeep, was around $10 billion U.S. But by then, our best ground-based telescope was computerized. It was over three times sharper, and over seven times cheaper. It was also far easier to maintain. Nor did we have to risk lives to do so. The same thing happened to costly satellite phones from the early 1990s when cheaper cellular phones showed up in the late 1990s. Many satellite-phone companies died. Investors lost billions.
Worldwide, the solar market, even on the ground, far less in space, isn’t even yet an infant; it’s still a fetus. And its strong dependence on state subsidy makes it fragile. Thus, in 2008 Spain cut its solar subsidies by about a third. The next year, the world market shrank from an expected 2.5 gigawatts to around 0.375 gigawatts. In 2009, Germany had our largest installed toolbox of solar power. After decades of state subsidies, it generated about 5.4 gigawatts of Germany’s power. Was that a lot? Well, yes and no. Compared to everywhere else, it was a lot. But it was also only about one percent of the country’s total electricity needs. So where did Germany get most of its power? Coal.
Further, orbital energy, like every other possible future energy option, would have to compete with our present energy suppliers—none of whom might be keen to welcome a new kid. Thus, in 2002 Exxon Mobil partnered with others to fund a new energy research effort, pledging $100 million U.S. Such funding levels made the news. But news reports failed to mention that $100 million was only about a day’s worth of Exxon’s profits. Also, that money was to be spread over a decade or more. So it amounted to less than three hours profit per year. Similarly, in 2006 British Petroleum pledged to invest $500 million U.S. in new energy research. But that was only about a week’s profits for BP—and, again, it was to be spread over ten years. In 2010 BP spent over $93 million on advertising alone. In what way are these companies serious about new energy research?
On the other hand, in the 1970s oil prices jumped and seemed likely to stay high for years. That scared our big oil companies. So they scrambled to invest in new energy research for real. That increased research, plus real conservation, plus new oil finds in Alaska and the North Sea, scared our big oil suppliers. So they rushed to cut prices. They realized that overly quick price hikes, or overly unstable prices, would only drive them out of business faster. The only sure way to kill competition was to both lower and stabilize oil prices.
That cycle of rising demand followed by rising prices followed by falling demand followed by falling prices will almost surely continue. Likely it will stop only when demand is too high and supply too low for anyone to alter. Since the 1980s, not even the planet’s biggest players drive that cycle. The market does—which means we, the cheapskates, do.
In the longer run, though, orbital power may still be in our future. Space flight today is like throwing eggs into a tree. To survive the toss, the eggs have to carry their nest with them. That means intricate and costly slingshots and extremely careful launches. But another way is to first build a nest in the tree, then launch the eggs.
To build stuff in space we need brains and hands up there. To get that, we fling big, finicky, costly rockets because we have to launch ourselves. Never send a human to do a bot’s job. Spacebots would need neither food nor air nor water—nor a ride home. Plus, if one blows up, so what? So if we could make them smarter and cheaper and more dexterous, staging missions could grow cheaper and easier. Lots of small launches could send up fuel and parts, plus more bots. Instead of everything having to happen in the blink of time before space travelers die, mission buildup could go on for years.
However, while spacebots may be in our future, that needn’t mean that we’ll bother building lots of them anytime soon. We can catch solar energy on the ground much more easily than up in space, so that’s where we’ll continue experimenting. Only when we have proven tech here are we likely to try it there.
Further, tossing eggs into trees is more exciting than tossing nests into trees. There’s always the chance that the eggs will break. So funding for egg-tosses far exceeds funding for nest-tosses. We’ll likely also continue to toss eggs if another exciting space race develops. We like horse races.
That will likely continue to be true unless nest-launch gets so cheap that we do it routinely. If that happens, we won’t just be using bots to explore space, we’ll also be using them to build in space. Likely we’ll first teleoperate them in orbit, as remote hands. Then, assuming they get smart enough, we could build ones that can build copies of themselves out of moon rocks and sunlight. If so, they could then go on to build orbital power plants for us out of more rocks and sunlight. Mostly what we would then be launching is knowledge, and what we would get back is energy.
Thus orbital solar energy might be in our future, but if it is, it may be at least as far away as 2040 or even later. So—probably—is fusion energy, as are all our other potential energy options. None are cheap enough or well-understood enough yet for us to deploy them widely soon. It doesn’t matter whether it’s space-based solar or ground-based solar. It’s irrelevant whether it’s photovoltaic or solar thermal. It’s unimportant if it’s wind, tidal, ocean thermal, or life-forms engineered to make oil. Until research on one of them nearly guarantees a big pile of money to somebody shortly—or a big edge to one state over another soon—investment will likely continue to be in dribs and drabs.
But as crude oil prices almost surely rise, so will other options grow more attractive. As we pour a little more money into each of them, we’ll learn how to make some one thing more cheaply. In other words, we’ll deploy them only when they pay, and not much before. That’s the trouble with the future. We often forget that it’s a birthday present that’s always marked ‘Some Assembly Required.’
Spirits from the Vasty Deep
Given the stigmergic effects of our present toolbox, what might be the near future of our materials and energy?
In 1884 in the United States, we crowned the Washington Monument with a 100-ounce pyramid. That pyramid, which had earlier been on display in Tiffany’s jewelry store in New York, was so prized because it was made of aluminum—a new, scarce, and precious wonder metal. It cost $16 U.S. a pound—the price of silver. Today, though, we use it to make throw-away soft-drink cans. In a little over a century, our supply of it per hour of work went up about 100-millionfold.
On the other hand, in 1845 in Britain, we saw coal tar—the liquid left after heating coal to make coke—as a smelly, sticky waste product. We couldn’t give it away. We couldn’t burn it or bury it, either—or, rather, if we did, we had an even worse problem. So we snuck out at night and dumped it in the nearest river. That stunk up the water and killed fish for hundreds of miles downstream. But as we learned more about it, we used it to make waterproof goods—first raincoats, then varnish, wood preservatives, and asphalt. Then we made colorfast dyes, then perfumes, cosmetics, vitamins, drugs, explosives. Then we figured out that if we mixed hexamethylene diamine and adipic acid—both extracted from the same smelly, sticky coal tar—we would get... nylon. By 1945, women were rioting to get more of the new wonder stockings, which were both cheaper and sheerer than silk ones. Millions of pairs sold out in days. In a century, coal tar had gone from something that we paid someone to cart away into a guarded resource.
As with our food and labor, our resources (that is, materials and energy) can change over time. They limit what we can do, but in the long run, don’t limit us because, like food and labor, materials and energy are related, via knowledge. Cheap energy plus knowledge can give us cheap materials—as happened with aluminum. Cheap materials plus knowledge can give us cheap energy, or yet more cheap materials—as happened with coal and coal tar. However, it’s hard to get knowledge unless we already have cheap energy and materials—and knowledge. Thus, in the long run, neither energy nor materials limit us. Knowledge does.
Knowledge has always limited us. When we were foragers in Iraq, we could have dug oil wells, but we had no idea that they might be useful. In Jordan, we could have built nuclear power plants, too, since we had uranium, but we didn’t know how. Instead, much later, Navajos and Utes in Colorado used carnotite (one of its powdery yellow ores) as body paint. And much later, Czechs and Germans in Bohemia used another of its ores, pitchblende, to glaze pottery. As foragers, then farmers, we were surrounded by resources that we today use, but we didn’t see them as resources. They were rocks and dirt.
It’s just the same today. Every day we walk past resources that once were vital to us. Even our bodies make resources that we could use. We could make phosphorus and ammonia from urine—or gunpowder from charcoal, sulfur, urine, and dung. Once upon a time, we did; but today we’ve forgotten how because there’s no need. We think so little of such resources that we pay someone to cart them away.
Our future will be just the same. All today’s power plants and cell phones and jet airliners were always here. Their parts were buried in the soil or floating in the sky, waiting for us to see through their masks. All the materials and energy that we today assemble into steel plows and breast implants and protease inhibitors were ready for us millennia ago. What we lacked was knowledge that such products were possible, and knowledge of how to make the tools to make the tools that we needed to make them. It’s certain that many future wonders are all around us today, too well hidden for us to see just yet.
That matters today because now we seem to be gearing up for a new phase change in materials and energy as we learn how to make machines at the molecular scale. Once we get down there we’re almost surely going to unmask all sorts of new things. Yesterday we learned how to make beer in vats. Today we’re learning how to make oil in vats. Tomorrow we may learn how to make meat in vats. Then may come fuel-plants that can harvest sunlight and carbon dioxide ten times faster than normal plants do. Maybe we’ll also make algae farms that live on methane, or ocean farms that give us both food and fuel. Such tools might also give us more control over the atmosphere—in case we ever decide to do anything about climate change other than talk about it. In such a future, things that we yesterday thought of as vital, like flint and obsidian, or that we today think of as irreplaceable, like oil and diamonds, we tomorrow may think of as garbage—and we’ll pay someone to cart them away.
But while knowledge changes us, that usually happens only after we inject it into our toolbox, and that takes time and money. Thus, a century ago, our streets smelled of dung and one day they may smell of ozone, but changing from one to the other won’t be cheap. Nor quick. Nor painless.
Today we batten on the planet’s oil the way ticks bloat on a dog’s blood. Our oil-fueled changes have been so vast and so swift that we now face serious problems. The list only starts with massive species loss. It includes forest loss, topsoil loss, water pollution, air pollution, and overuse of antibacterials, fertilizers, hormones, and pesticides. The planet has long known that we’re a high-maintenance date. For instance, in the last 50 or so years we’ve thrown away over a billion tons of plastics. Most don’t decay, so nearly all of it is still there—in the oceans, in landfills, in the stomachs of birds and fish who are shortly about to die from it. That can’t go on forever.
Of course, we might decide to cut back on meat and cars. Or we might decide on a crash program to develop new fuels. But, short of some big disaster, both futures seem about as likely as us volunteering to pull our fingernails out. Likely, any such change might well cost as much as a big war and we, the cheapskates, almost never spend that kind of money willingly—except for a big war. Thus, in 2009, the United States government spent $3 billion on energy research. But that same year it spent $77 billion on weapons research. As of 2006, and worldwide, we burned about a thousand barrels of oil every second, and our oil investment is (allegedly) upwards of a trillion dollars U.S. a decade. It’s unlikely that some piddly research grant worth a couple million dollars a year could overturn so heavy an applecart. We don’t just have our snouts in the oil trough; we have our front trotters in there, too.
The stigmergic effects of our present toolbox are just too strong for quick turnaround. Any new transport fuel would be trying to overturn well over a century of investment and research and development on gasoline and diesel fuel use in internal combustion engines. Trying to go against that would be like trying to turn a supertanker. It can be done—but not cheaply or quickly. So, instead of spending many billions on research now, we’ll almost surely continue to spend a few trillions on occasional wars and other patches and bandaids to preserve our current fuel synergies for as long as we can.
But no matter how much blood and treasure we spill, in time the price of crude oil most likely will rise. As it does, we’ll divert a few more billions to other options. We’ll come across a small win here and a little gain there, until other options become cheap enough to use in niche markets. Then those might spread. Even then, though, oil will still have the cheapskate’s advantage because of its huge toolbox. Then might come some sort of crisis—perhaps abrupt climate change, or our next world war. Then we’ll panic. Investment will then more quickly move into a new fuel toolbox. Stigmergy likely means that turning point may well be at least 2040 (unless we solve the ‘make-big-reusable-batteries’ problem between now and then) or perhaps even further into the future (especially if we crack the ‘make-cheap-oil’ problem between now and then).
In sum, stigmergy means that we might well start changing our materials fairly soon, because less has to change, and our factories are already automating, so it would be easier to change them, but we’re much less likely to change our energy supplies anytime soon, because a lot would have to change. If we don’t solve our transport fuel problem soon, we’ll probably have to go back to slower or more limited-range personal vehicles, and that will place all sorts of limits on trade and settlement.
However, stigmergy, and the recursion it enables, also means something else, too—if change ever does start, along any direction, it will be hard to stop.
We started into our industrial phase change in at least four waves. First came the steam engine. That gave us relocatable power. We could then power machines to make things no matter where we were. No longer did we have to site our manufactories next to swift-running rivers. Then came the railroad. That linked us. No longer did nearly all of us have to be self-sufficient farmers. As manufacture and transport and trade spread, more regions specialized, urbanization grew, and division of labor multiplied. Then came precision parts and assembly lines, which led to mass-producing factories. No longer did we have to rely on scarce and scattered skilled labor. We could concentrate and specialize our labor in single buildings. We could then use specialized power tools anywhere to make anything and trade them widely. Then the fourth wave happened (largely) when we mastered electricity and magnetism and used them to make machines that we fueled with hydrocarbons. All four waves together meant that many more of us than before had cheap food, labor, materials, energy, goods, and services. We started to break out of the farming bottle.
That phase change didn’t start when it did because we planned it. It happened when we, without even knowing it, first blundered into a way to build the main pieces of a huge dynamo of change. That dynamo was far larger than the steam engine, or the railroad, or the factory, or the particular kind of electrical machine that we today call a dynamo.
For it to come to exist, we first needed to amass much knowledge—and that knowledge had to be both reliable and written, so that we could both trust it and spread it. Over many countries, and many millennia, tens of thousands of us had to have been motivated to create, amass, recombine, test, and spread that knowledge.
But that wasn’t enough. Just for that knowledge to stay alive, far less grow, relatively many of us needed to be able to read it. Then, for it to keep growing, a fair number of us—not just our few priests and wizards—needed to be able to add to it.
As well, we needed to have amassed many specialized tools and skills. We also needed to have amassed a lot of capital—which isn’t mere cash but frozen human energy—gathered at least partly from our enslaving of each other. We also had to have amassed enough financial and trade tools, like banks and bonds and ships and such, to put that capital to work building new things or finding new trade deals. Plus we had to have big enough cities to amass our brains and hands to thus build all those tools.
Absent any of those pieces in the long pinball game of history and our new dynamo might not have come to exist when it did—or perhaps at all. In the event, however, all those pieces locked themselves together into a dynamo. As it spun up, it stigmergically added to our knowledge, skills, tools, and capital.
It’s still doing so today. Rapid income growth began in Britain in the late 1700s. That spread to the United States by the early 1800s. By the late 1800s, France and Germany and several other countries in Europe, and its transplants, also spun up, as did Japan. Then came two hot world wars in quick succession, followed by a long cold one as two giants arm wrestled for planetary dominance. Then, by the mid to late 1900s, the same rapid income growth spread to other parts of Asia as places like South Korea and Singapore took off. By 2010, our fastest income growth was in China and India, where more than one in three of us lived. Our previous big growth spurt, from 1860 to 1910, was largely limited to our presently rich countries, but our latest spurt is the broadest that our species has ever undergone. We seem to be entering industrial adolescence.
Adolescence, though, isn’t just an age of growth spurts, deepening voices, and training bras. It can also be a time of peer pressure, hopeless crushes, awkward groping, and perhaps even self-destruction. Today, our labor continues to specialize and divide, bots are rushing into our factories, and our factories are hopping all over the planet seeking our cheapest labor pools. Suppliers, shippers, advertisers, and retail outlets are growing more global and more swappable. Inventory is growing more just-in-time. Jobs are growing more transient, more global, more swappable. Maybe global metaconcerts might be next. Many of our lives are changing as our computation and communication tools cheapen and spread. ‘Mass production,’ in every sense of the term, is spreading.
From the mid 1800s on, our companies competed to make more machines to aid, then to replace, our manual labor. As machines chased us out of each field, we stopped being their competitors, becoming instead their operators and maintainers. As we fled from the fields they bested us in, we squeezed into fewer, more skilled fields. But that only raised competition there. Also, after a while, they pursued us even there. So as our recursive machine base grew, even our skilled labor began to fall in value. Over time, taking pride in handwork became commercially valueless. So cultivating skill in craftwork became art. (And what happened to our muscles back then may soon happen to our brains.)
Then, as whole nations competed, the same thing began happening to entire industries. Knowledge was flowing from us to our increasingly intricate machines. So differences in skill between us no longer mattered as much as it once did. In more and more fields, our skills became so mechanized to fit the machine that our tools began replacing us even there. More and more of us, whether skilled or not, started becoming as swappable as the parts our tools could now make. Thus, at first, single jobs evaporated, then whole fields began to evaporate.
So just as we started turning into operators when machines began to replace our muscles, over time we started turning into planners as machines begin to replace even our brains. As a result, today we’re always running to find money we don’t yet have, to build factories we haven’t yet finalized, to make tools we haven’t yet perfected, to run things we haven’t yet fully designed, to sell to markets we can’t yet imagine. And next year we’ll do it again—except faster.
In short, our phase change into industry didn’t happen long ago. It’s still happening. We’re now at its hump point as change maximizes across the planet. We keep shortening the distance between idea and reality, moving toward a day when just one of us can have an idea, snap our fingers, and make it real. But what would that idea be? To help whom? To harm whom? And how would it network with every other idea that everyone else is just as busily snapping their own fingers about at the same time? Adolescence can be an exciting time, but it’s also often a moody and unsure time—even more so now as we bumble and stumble and muddle into an increasingly unsteady future. Like Prospero, we have worked, and are still working, rough magic to achieve a design that we cannot foresee. Are we turning into something rich and strange? Or are we about to do something that will break our staffs and drown our books?